Purpose
The purpose of this brief overview of the Planetary Boundaries framework is to provide a summary of the framework and the goal that it tries to achieve. In addition, I hope the information provided here serves as an introduction to inspire the reader to examine the strategies, regulations, and practices in their industry, business, organization, and personal life and reform what needs to be reformed accordingly. Once you become familiar with this framework’s scientific approach and findings, you will realize the compelling necessity for a net positive approach to all these environmental shenanigans.
Holocene
According to the geological time scale, the Holocene is an epoch (a subdivision of the Quaternary period) which started around 12000 years ago. During this epoch, the Earth system processes have evolved and reached relatively steady state conditions with the functionality to regulate negligible environmental perturbations to sustain a state that has been stable enough to allow human evolution, civilization, and development. Up to the onset of the industrialization era around 1880 AD, the anthropogenic perturbations to the environment had been sufficiently negligible for the Earth’s regulatory processes to stabilize the Earth’s subsystems/processes in conformity with the Holocene state.
The Earth’s subsystems and processes are in direct, indirect, and positive/negative feedback-loop interactions, all of which could be linear or non-linear. Therefore, any perturbation to the state of any of these processes affects the other processes with a time lag. In other words, significant changes to one subsystem would eventually trigger a perturbation in other subsystems as a function of time. On the other hand, suppose the perturbations in the first subsystem are regulated in a timely manner. In that case, other affected subsystems/processes will continue to be involved through linear and non-linear feedback-loop effects for some time before stabilizing. However, suppose the impacts become too large for the processes to regulate. In that case, the affected subsystem will deviate from its current trajectory and transition to a new state outside the Holocene-like state, possibly irreversibly. An example of this scenario is the Antarctic ozone hole that was developed unexpectedly due to the extensive emissions of CFC compounds. A substantial chemical influx of one process led to the failure of another process.
Regulatory Functions
The regulatory functions of every subsystem have evolved to sustain the Earth system as a whole in the Holocene-like state on a long-progression trajectory into the next state. This progression is steady enough to adapt the entire system and its species adequately. Although the Holocene is a transient state spanning thousands of years, forcing the Earth to exit this state too abruptly will inevitably compromise the integrity of all its subsystems, such as the biosphere and its living species.
According to fossil records, the background extinction rate during the last mass extinction event (five in total) is estimated to have been 0.1-1 species per million per year for marine life and 0.2 to 0.5 for mammals. The current extinction rate is estimated to be between 100-1000 (as per 2015 assessments, possibly higher now) species per million per year. With regards to humans, the impact will be inadvertently deleterious on development and quality of life if not on our existence as species. The way of life we have come to know since the industrialization era will no longer be recognizable to us and will be critically compromised for the next generations if the concerted anthropogenic adverse changes to the Earth’s processes persist.
The Framework
What is the Planetary Boundaries framework, and what are its critical Earth system processes?
In 2009 a group of scientists published (1, 2) a proposed framework for estimating the global state of the Earth’s biophysical and biochemical processes/subsystems to be used as a guideline to determine safe operating criteria for human development. The framework encompassed nine processes, seven of which had the associated quantifiable control variables with proposed boundaries based on pre-industrial or Holocene-like baseline values. The measured observables of these control variables suggested whether and to what degree we had deviated from the baselines.
An update to the original framework (1, 2) was published in 2015 (3). As per the 2015 publication, the nine components of the framework are based on nine Earth subsystems/processes: 1- climate system, 2- biosphere; 3- land system; 4- freshwater system; 5- biochemical cycles; 6- ocean system; 7- atmospheric aerosols; 8- stratospheric ozone system; and 9- novel entities.
The corresponding components for the Planetary Boundaries framework are 1- Climate Change; 2- Biosphere Integrity (Rate of Biodiversity Loss in 2009)- this process is composed of two components, Functional Diversity and Genetic Diversity; 3- Land System Change; 4- Global Freshwater Use; 5- Biogeochemical Flows (Biochemical flows, interference with Phosphorous and Nitrogen cycles in 2009); 6- Ocean Acidification; 7- Atmospheric Aerosol Loading; 8- Stratospheric Ozone Depletion; 9- Novel entities (Chemical Pollution in 2009).
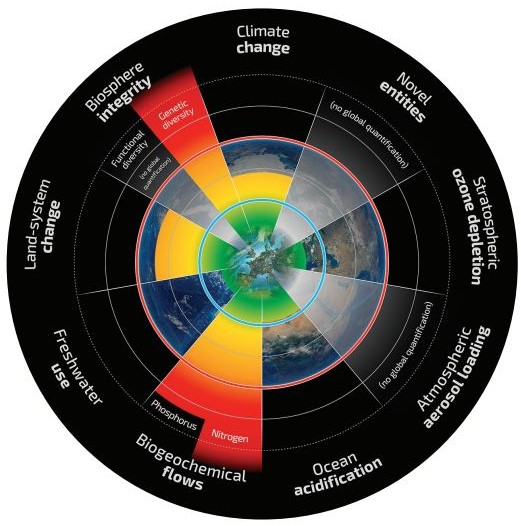
Since the onset of the industrialization era around 1880 AD, human development has led to anthropogenic perturbations to the Holocene state, identified to be significant and too abrupt for the Earth system and its subsystems to adapt accordingly to stay in the Holocene-like state. These human-induced extremes are destabilizing the buffering capacity of the Earth’s processes, altering critical bio-geophysical and bio-geochemical cycles. The principal goal of constructing the framework’s proof-of-concept was to propose a set of standards to confine, constrain, and guard-rail human development and its environmental impact within a safe operating space- a space in which Holocene-like conditions are not violated irreversibly.
The control variables for each process are chosen as a quantitative representation of the critical aspects and functions, measuring quantities such as material concentrations or flow rates. These variables are not unique or absolute; however, there is a strong consensus about them in the community. The threshold refers to the upper boundary of the control variable that, if crossed, the subsystem/process has exited the uncertainty zone into the high-risk zone.
The proposed boundaries for control variables are derived based on a three-fold strategy/assumption:
1- Due to the scale of these subsystems, the boundaries should be set far enough from thresholds to account for the overshoot of control variables.
2- Due to the complexity of the processes and their interactions, the boundaries should be set far enough from the thresholds to allow accurate updates of the state of the processes. For instance, when the Climate Change control variables cross their thresholds (which the radiative forcing control variable has- by a large margin), their impact on other control variables (processes) will come into effect with a time lag. However, if the width of the uncertainty zone is defined adequately, we can update and adjust other control variables accordingly and promptly.
3- The third reason is to allow ample time to implement and monitor regulatory and governance actions because we are not as proactive as we think.
Crossing the boundary moves the corresponding subsystem/process from the safe operating zone into the uncertainty zone. Transgressing the threshold (exiting the uncertainty zone) indicates the subsystem/process has entered a new phase with unpredictable effects on the Earth system and the other eight components of the framework. The interdependence of these interacting processes implies that forcing one into the uncertainty or the high-risk zone may force others into their respective risk zones earlier than predicted and change the initially estimated boundaries for other control variables. Once a process enters the high-risk zone, the transition is potentially irreversible, and the probability of it destabilizing the Earth system is very high, especially if it is a core process such as Climate Change or Biosphere Integrity.
There are various models of planetary boundary frameworks, some proposing global-scale control variables and thresholds and some proposing boundaries based on a local scale (e.g., intensified local chemical influx into the environment such as agricultural zones with extensive use of fertilizers, large outflux of wastes from mining and fossil fuel processing, and chemical facilities), regional scale (large scale conversion of natural lands to crop-lands and deforestations such as the Amazon forests); and global scale (atmospheric CO2 concentration). In addition, researchers have been working towards creating more precise continental- and regional-based frameworks to facilitate and optimize the development, implementation, and monitoring of governance, policy-making efforts, industry practices and accountability at regional/local levels.
Two of the nine processes are considered core processes, meaning they have the most potent interactions with other processes and the most critical impact on the Earth’s Holocene-like state. Climate Change and Biosphere Integrity. The following sections will explore each process, starting with the core processes.
Climate (System) Change
Core Process
Overview
The climate system is a complex system composed of five subsystems, the atmosphere, hydrosphere (water, vapour, etc.), cryosphere (ice, permafrost, etc.), biosphere (living organisms), and lithosphere. These five components are in constant interaction. The statistical characterization of the climate system is commonly referred to as the climate. Climate represents the long-term-large-scale behaviour of weather, in other words, the global weather in the long term. The climate is a strong function of its components, such as ocean and atmospheric currents and impacts the functionality of all Earth’s bio-geophysical and bio-geochemical processes.
The climate system is strongly affected by the energy budget above the Earth’s surface. The energy budget is regulated through radiative influx and outflux at the top of the atmosphere. Therefore, the climate system will change if the outgoing energy is smaller than the incoming energy. In addition, the energy balance is effectively influenced by atmospheric gases through radiative scattering, absorption, and reflection processes. Greenhouse gases (GHGs) such as CO2 and methane enhance the heat-entrapment process, leading to the increased global mean temperature, hence the change in the climate system. These gases have different atmospheric lifetimes and radiative absorption/scattering/reflection capabilities. In the planetary boundaries (PB) framework context, Climate Change is a core Earth process that can deviate the Earth system from its current Holocene-like state due to its substantial impact on other subsystems. Although the biosphere is a subsystem of the climate system, the integrity of this subsystem is examined as a separate PB component.
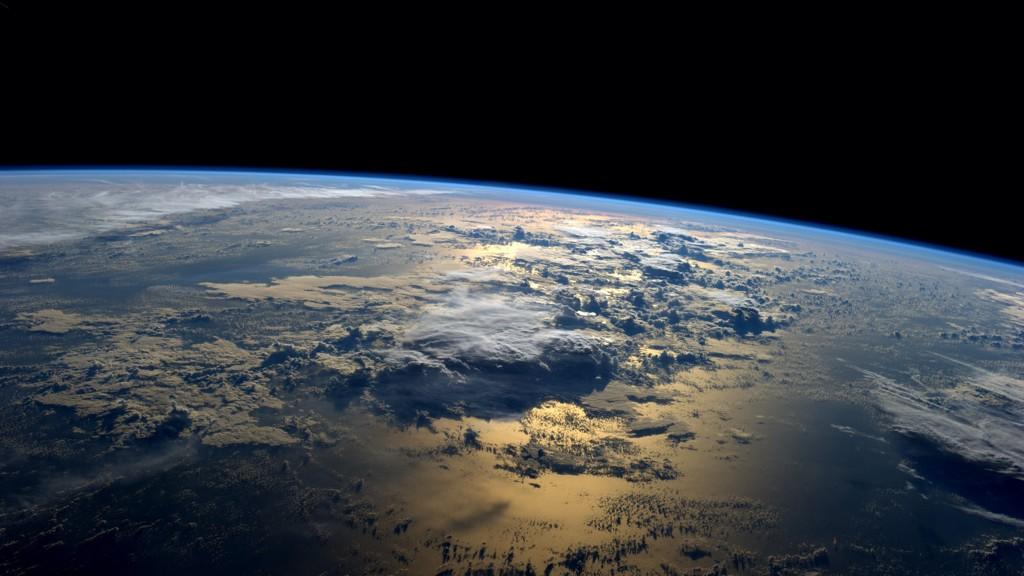
Climate (System) Change
Core Process
Control Variables
Two control variables are selected for Climate Change, and their boundaries are proposed based on pre-industrial baselines.
1- Global mean atmospheric CO2 concentration (P.P.M.V., parts-per-million-per-volume). CO2 is chosen based on its long lifetime in the atmosphere and is the largest anthropogenically emitted GHG by volume; however, global warming’s effect on the rapid melting of permafrost and the tremendous release of trapped methane may increase the role of methane as a GHG significantly.
The CO2 boundary is set at 350 ppm. The uncertainty zone initially had been set to the [350-550] ppm range. However, based on more recent studies presented in IPCC reports, the range has been narrowed to [350, 450].
The current value as of Feb 2023 (https://climate.nasa.gov/) is 419.49 ppm. Around 20 ppm increase since 2014, possibly entering the high-risk zone in the next few years.
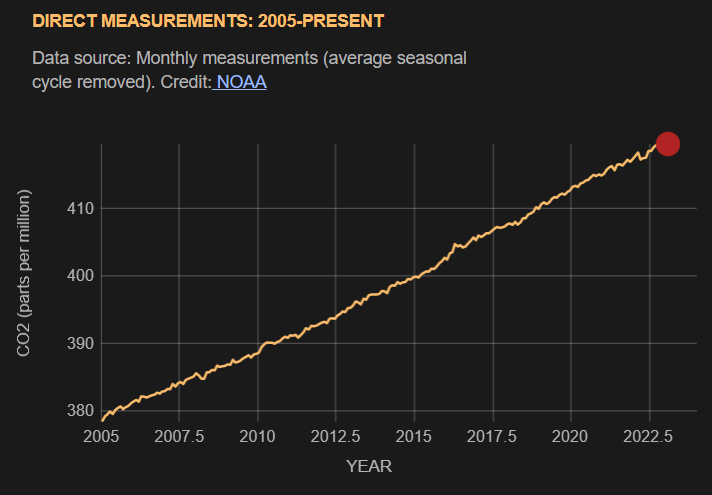
Climate (System) Change
Core Process
Control Variables
2- Radiative forcing at the top of the atmosphere (watts-per-meter-squared)- the incoming radiative energy at the top of the atmosphere per unit surface area, per unit time, minus the outgoing. This control variable is more inclusive and representative of climate as a system-in-change. It represents the effects of all GHGs, aerosols and other anthropogenic gases that trap energy above the Earth’s surface. The boundary is set at +1 (watts-per-meter-squared). The uncertainty zone is set to the [+1, +1.5] range. The current value is estimated between 2.5 and 3 (Wm^-2), which has exceeded the threshold.
Current Status
The climate system is on the verge of entering the high-risk zone.
Climate Change – Interactions with other processes
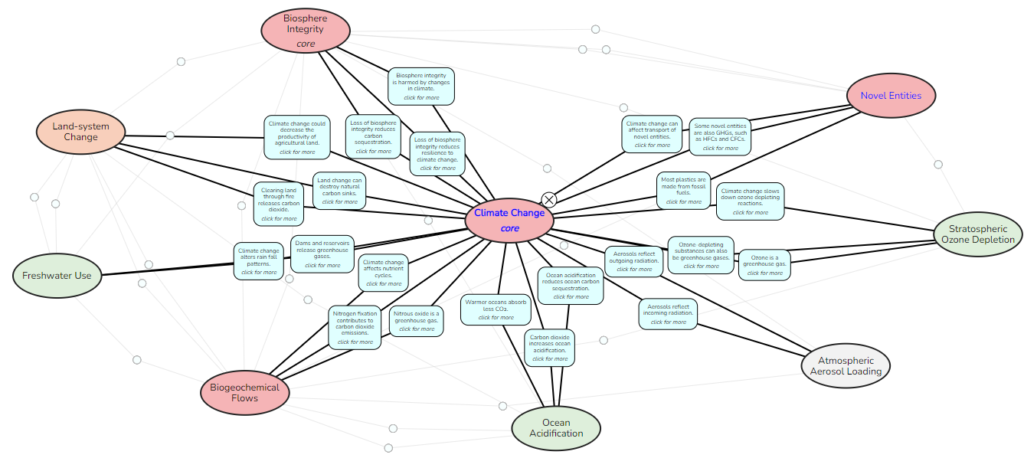
Biosphere Integrity
Core Process
Overview
Biosphere is one of the five subsystems of the climate system. Biosphere represents all living organisms, and the scale of its impact on and from all other Earth subsystems and processes makes it a core process. The integrity of the biosphere is a component of the PB framework which studies the changes to the biosphere system and its role in the Earth system stabilization. The Biosphere Integrity process is composed of two components:
1- Genetic Diversity, which examines the impact of the loss of genetic diversity.
2- Functional Diversity, which aims to examine the impact of the loss of functional diversity. Functional diversity refers to the diverse functional role of organisms in the system. Those going extinct had functions in their ecosystem, and those functions will be gone. Loss of functional diversity tries to quantify the impact of lost functions by understanding their ranges, values, and distributions in a biota or ecosystem (6). Although both components of this process are complex and fundamental, the quantification of the functional diversity has been challenging enough that, to this day, there still needs to be a proposed control variable.
Biosphere Integrity
Core Process
Control Variables
Genetic diversity provides the biosphere’s long-term potential/capability to coevolve, evolve, and adapt to natural and anthropogenic disturbances to and from other Earth systems and processes. For the first-order best estimate of the biodiversity integrity, the phylogenetic species variability (PSV) was to be used as the control variable. PSV, in conjunction with functional diversity, could provide very inclusive and comprehensive control variables more sensitive to anthropogenic disturbances to the biosphere accurately; however, due to a lack of available global data for PSV and functional diversity, the loss of genetic diversity was an alternative interim control variable, and it is measured as the number of extinctions per million species per year.
As mentioned earlier, the background extinction rate during the last mass extinction event is estimated to have been 0.1-1 species per million per year for marine life and 0.2 to 0.5 for mammals. A recent estimate of the extinction rate is between 100-1000 (possibly higher now) species per million per year. The current status of this control variable places the Biosphere Integrity process in the high-risk zone. The impact of this core process in the high-risk zone and the Climate Change on the verge of it will be detrimental to the entire Earth system.
Biosphere Integrity- Interactions with other processes
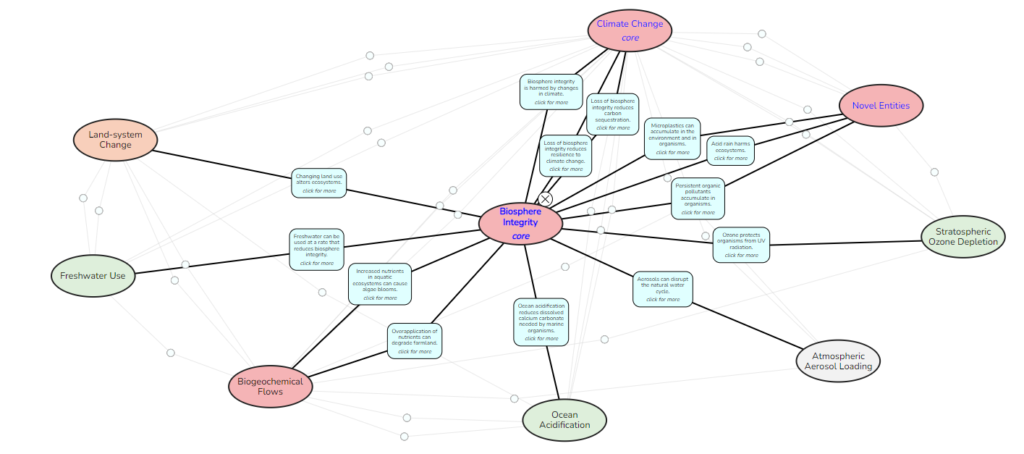
Land System Change
Overview
Terrestrial biomes include forests, savannas, grasslands, woodlands, shrublands, tundra, etc. The land system refers to the sum of the above subsystems. The change in the land system has become one of the PB framework processes in order to examine the interaction between the bio-geophysical processes of land systems and other processes such as climate regulation, water cycles, and energy balance.
Control Variables:
Initially, the control variable had been selected as the percentage of global land cover converted to cropland. As per the 2015 publication (3), the new control variable is the remaining forest cover. This change was due to the stronger role of the three major forest biomes (tropical, temperate and boreal) on the coupling interaction between land and climate systems. There are two boundaries set for two different forests (3); however, for simplicity, one boundary is introduced here, which is the global area of forested land as a percentage of original forest cover before the industrialization era. The diagram (source: https://applets.kcvs.ca/PlanetaryBoundaries/) shows the current state and its declining trend since 1990.
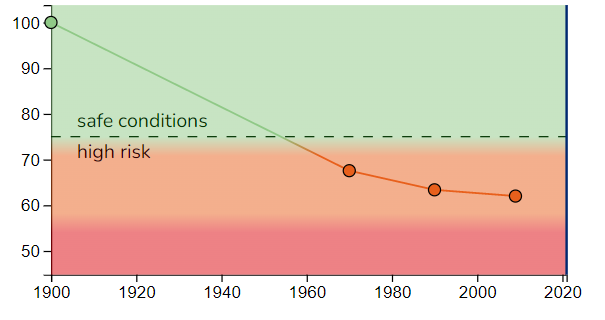
Land System Change – Interactions with other processes
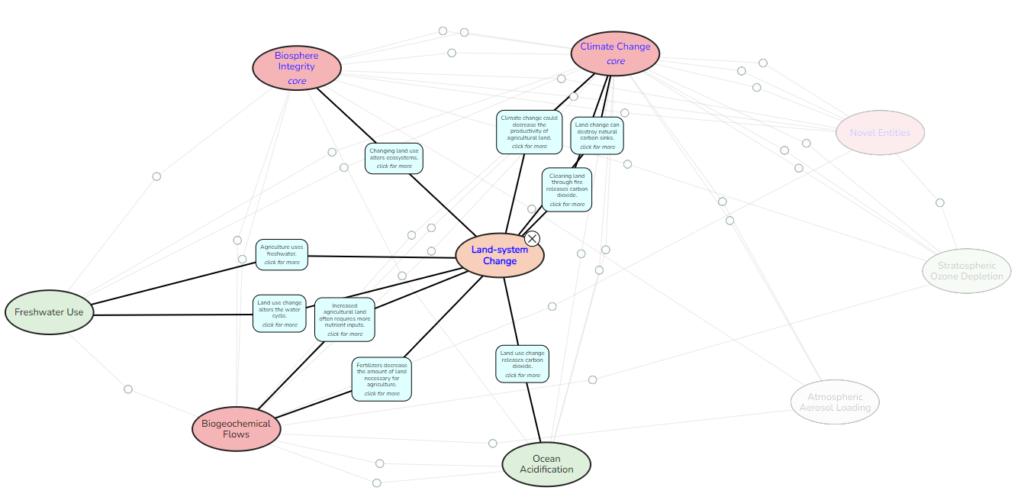
Freshwater Use
Overview
The freshwater system refers to the so-called blue water resources such as lakes, rivers, reservoirs, and renewable (in fact, not renewable at the current fast extraction rate) groundwater. The global manipulations of this system pose the following impact on other subsystems and processes (1):
1- The loss of soil moisture resources due to deforestation and degradation of other natural land systems, which comprise biomass production and natural carbon sequestration processes.
2- Overusing runoff volumes and disturbing the patterns threaten the regional and local clean water supplies, leading to security issues and widespread conflicts.
3- Posing negative impacts on climate regulation due to a decline in moisture feedback of vapour flows. Disturbances to the climate system lead to an immediate change in the regional and local precipitation patterns.
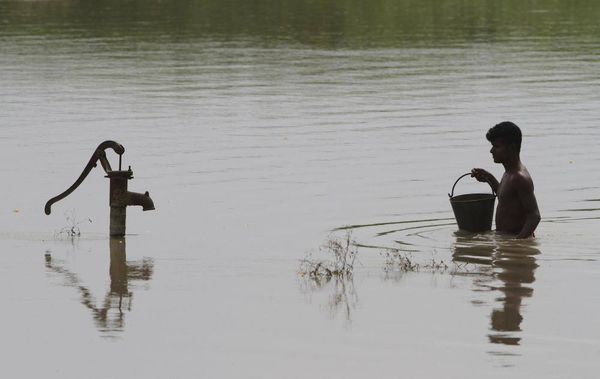
Freshwater Use
Control Variables
The global use of freshwater in units of cubic kilometres per year is selected as the control variable, and the boundary is set at 4000, with the uncertainty zone in the range [4000, 6000].
Current Status:
The current value for the global use of freshwater per year places this process in a safe zone for now. However, it is imperative to note that the global state of freshwater availability is irrelevant to its local and regional states. At local and regional scales, we have failed to provide clean water to hundreds of millions of people in many regions worldwide at every level. As a result, clean water scarcity, crisis, and related conflicts are rising in those regions, especially in Africa, West and Central Asia. In other words, the freshwater process has entered its high-risk zone locally and regionally.
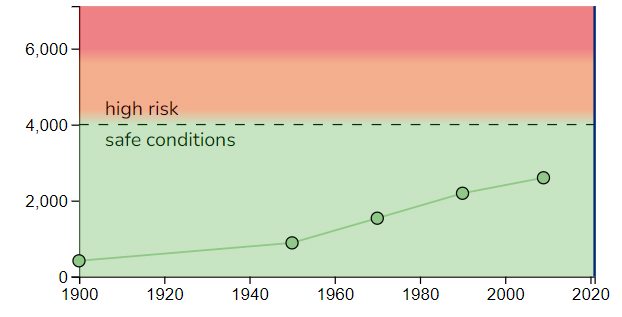
Freshwater Use – Interactions with other processes
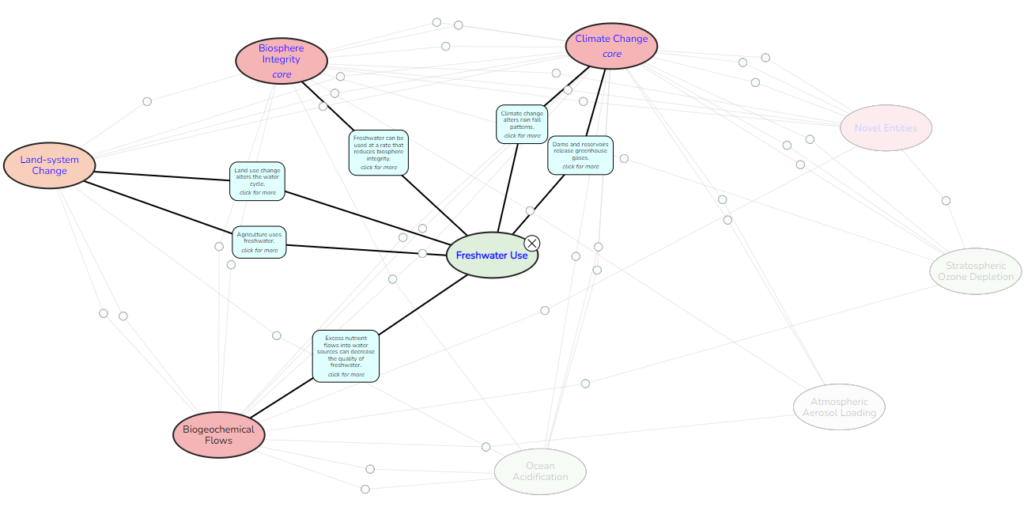
Biochemical Flows
Overview
Biochemical flows are the connecting pathways between the environment and living organisms. The natural nitrogen and phosphorus cycles are disturbed by local and regional-scale agricultural and industrial interferences, prominently through fertilizer production and application.
The conversion of atmospheric nitrogen to reactive nitrogen due to agricultural and industrial processes is now greater than all of Earth’s terrestrial processes combined. Four primary processes are responsible for the conversion of atmospheric nitrogen to its reactive derivatives: 1- industrial fixation of atmospheric nitrogen to ammonia (~80 Mt N yr-1); 2- agricultural fixation through the cultivation of leguminous crops (~40 million tons of nitrogen per year); 3- fossil-fuel combustion (~20 million tons of nitrogen per year); 4- and biomass burning (~10 million tons of nitrogen per year).
Much of this reactive nitrogen enters the atmosphere and precipitates back into the terrestrial biosphere, waterways, and coastal areas. Nitrogen fixation through processes such as Haber-Bosch is energy-intensive and requires methane for processing. Subsequently, it emits large amounts of CO2. In addition, byproducts such as nitrous oxide are potent greenhouse gases contributing to the climate change process.
Similarly, most phosphorous produced ends up in the aquatic systems rather than being absorbed by the crop plants. The high concentration of phosphorous in aquatic systems leads to the growth of algae, and the decomposition of algae requires oxygen, which in effect, takes up the available dissolved oxygen and starves other oxygen-dependent organisms. Interference with natural biochemical cycles has an adverse impact on the biosphere system.
Biochemical Flows
Control Variables
1- Nitrogen fixation rate (Tg nitrogen per year).
2- Phosphorous regional flow from fertilizers into erodible soil and from freshwater system to ocean system globally (Tg P per year).
Both control variables have transgressed their thresholds. As a result, this PB process has entered the high-risk zone.
Biochemical Flows – Interactions with other processes
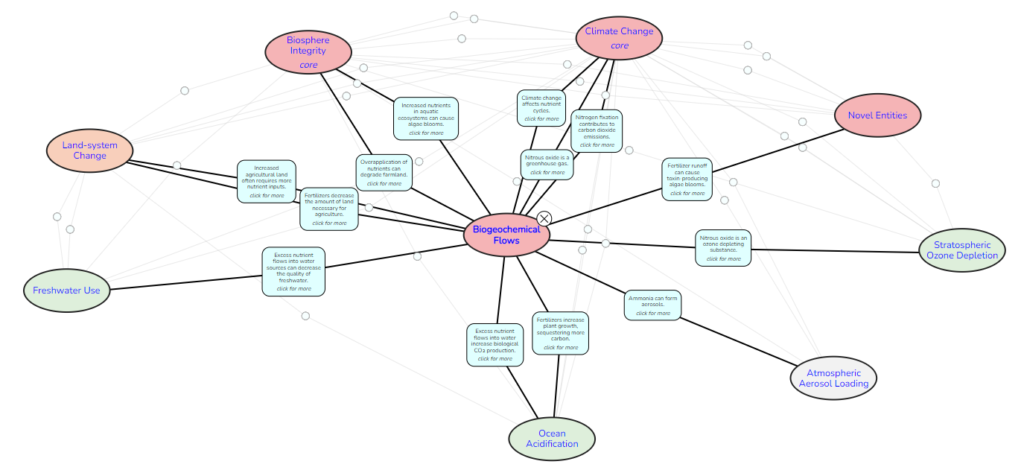
Ocean Acidification
Overview
The Holocene-like state has regulated the mean global acidity of the surface ocean system in such a delicate way that marine organisms are able to thrive. However, the organisms that process carbonate ions to construct their skeleton and protective shell structures are highly susceptible to the saturation state of aragonite (a form of calcium carbonate formed by many marine organisms). When the saturation state of aragonite drops below 1, aragonite dissolves.
The ocean system is a natural carbon sink. Currently, it absorbs around 25% of atmospheric carbon emitted through anthropogenic sources. The dissolved carbon forms carbonic acid, and this anthropogenic addition of carbonic acid to the oceans reduces the ocean surface’s pH, increasing global surface mean ocean acidity and compromising the marine component of the biosphere (a core Earth system process). As a result, the mean acidity (concentration of hydrogen ions) of the surface ocean waters has increased by 30% compared to the pre-industrial level.
Ocean Acidification and Climate Change processes have a common adversary- the atmospheric CO2 concentration. Therefore, climate Change, Ocean Acidification, and Biosphere Integrity processes of the PB framework are in strong interactions through the atmospheric concentration of CO2.
Ocean Acidification
Control Variables
The saturation state of aragonite of mean surface ocean waters has been selected as the control variable for this process. The boundary (set at 2.75) is based on sustaining 80% of its pre-industrial baseline (3.44), and the range of the uncertainty zone is set to [80%, 70%] of the pre-industrial value. The diagram shows the declining trend of the percentage with respect to the baseline (source: https://applets.kcvs.ca/PlanetaryBoundaries/).
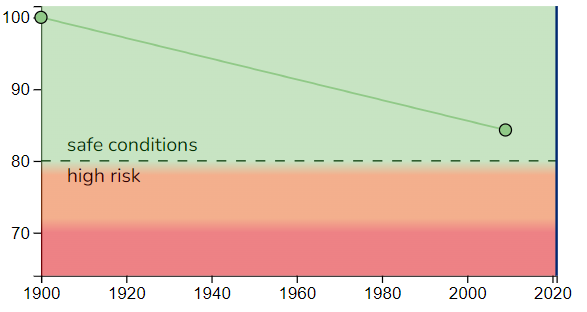
Ocean Acidification – Interactions with other processes
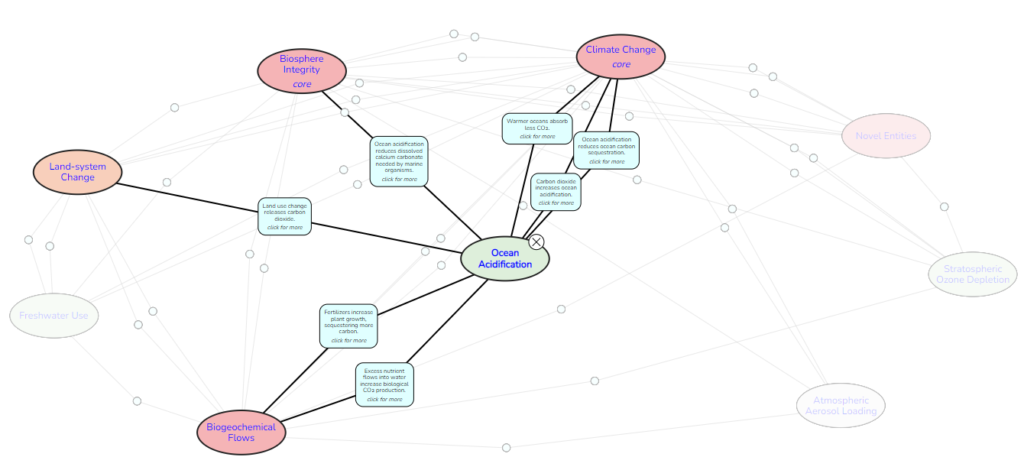
Stratospheric Ozone Depletion
Overview
Stratospheric ozone (O3) filters the incoming harmful UV radiation from reaching the Earth’s surface, and the thinning of this layer compromises the integrity of the biosphere system, altering the genetic and functional diversity. Skin cancer in humans is one of many examples.
The gradual rise in the concentration of ozone-depleting substances such as CFC (chlorofluorocarbons) and their interactions with polar stratospheric clouds changed the dynamics of the Antarctic stratospheric system. The unexpected result was the local disappearance of ozone from the lower stratosphere during the Austral spring (the season of spring in the southern hemisphere during which the greatest amount of ozone is lost, this generally begins in September).
Swift actions that came out of the Montreal Protocol led to the elimination of ozone-depleting substances, resulting in the decrease of tropospheric concentration of these substances and the onset of the self-recovery process of the stratospheric ozone layer.
The unexpected depletion of ozone proved the sensitivity of Earth system processes if they cross their thresholds. Again, once a process crosses its threshold, its stability and role in destabilizing other processes would be highly unpredictable, if not irreversible.
So, if the ozone hole is recovering and the problem is solved, then why is it still one of the planetary boundary framework processes?
When the concentration of greenhouse gases in the atmosphere increases, less radiation energy is reflected back to the stratosphere. In other words, the stratosphere cools down, resulting in the increase of polar stratospheric clouds. When this happens over the northern polar region, the ozone hole starts to develop over the northern hemisphere. Wouldn’t that be an apocalyptic scene?
Stratospheric Ozone Depletion
Control Variable
The control variable is the stratospheric ozone concentration in Dobson units (DU); its pre-industrial level was estimated to be 290 DU; the boundary is set at less than 5% reduction from the pre-industrial level, and the uncertainty range is set to [5%, 10%] reduction. The measurements of the variable are done over mid-latitude stratospheric columns away from the poles. The goal is to keep the values at or above 275 DU.
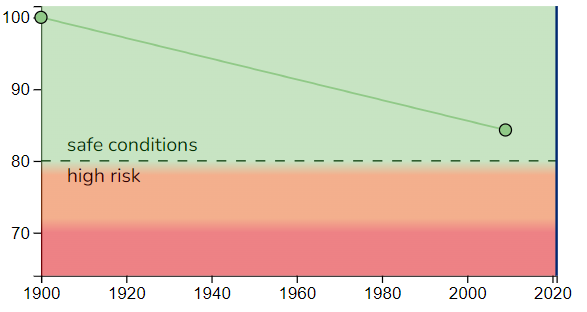
Stratospheric Ozone Depletion – Interactions with other processes
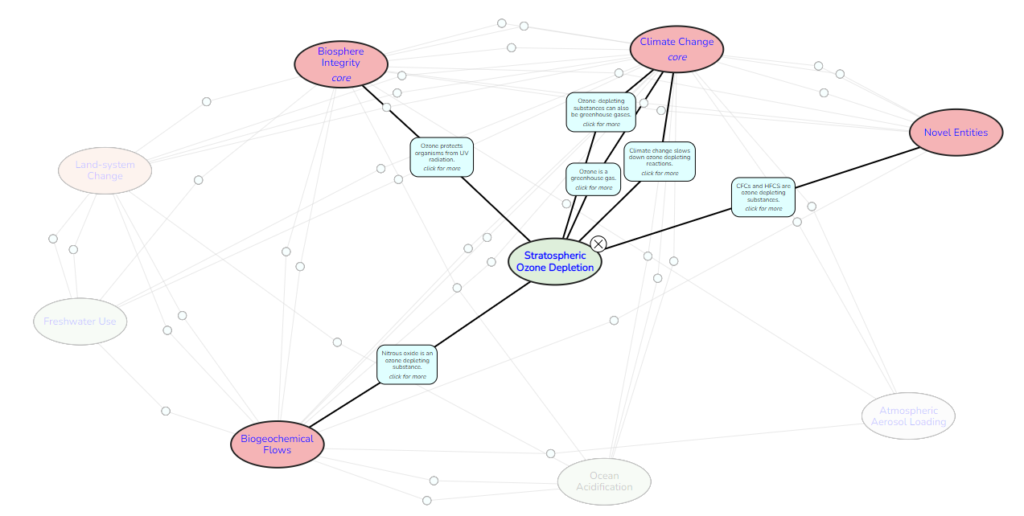
Novel Entities
Overview
Novel Entities (NEs) include chemical compounds that never existed in the environment, constructed and emitted intentionally or unintentionally through human activities. These toxic pollutants include heavy metals, nuclear wastes, persistent synthetic organic compounds, CFC, plastics, etc. These pollutants have potentially irreversible adverse effects on all living organisms in the biosphere system through altering reproduction rates and genetic modification.
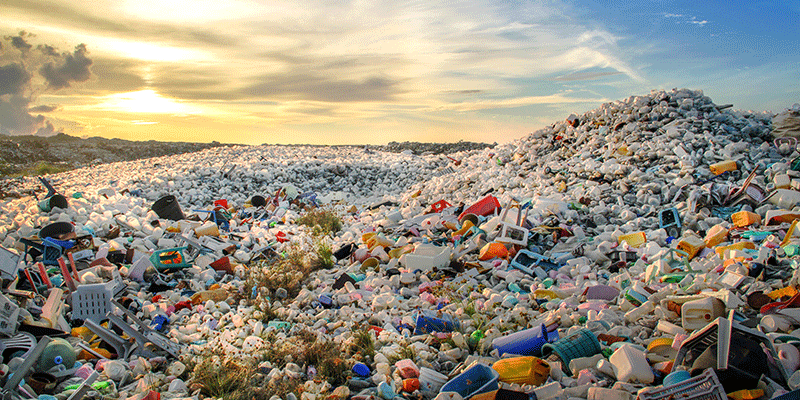
Novel Entities
Control Variables
The notable difference between this PB process and others is that there is no pre-industrial baseline to use as the background level. In addition, the added complexity arises because there are many compounds to account for. This has made it incredibly challenging for the researchers to propose control variables and thresholds based on a background baseline. This PB process had no defined control variable as of 2009 (1, 2) and 2015 (3) research findings. However, In 2022 a group of scientists proposed three overlapping categories with potential control variables. These three categories are based on: 1- the production of NEs, 2- the release of NEs, and 3- the unwanted impact of NEs on the Earth system.
Each of these categories proposes potential control variables assessed based on three factors:
A- Feasibility. How easily can the control variable be quantified?
B- Relativeness. Can the effects of the control variable be linked to other Earth system processes?
C- Comprehensiveness. Can the control variable represent the entirety of the impact of all NEs at the planetary scale?
The proposed control variables in each category are as follows:
1- Control variables based on the production scale of novel entities:
A- Production of plastics. For example, the production volume of plastics is measurable and representative of a large portion of novel entities. However, its degree of impact on other Earth system processes needs to be quantifiable.
B- Production of chemical compounds.
C- The share of chemical compounds with safety data or regulatory assessments.
2- Control variables based on the release of novel entities:
A- Release of plastics.
B- Release of chemical compounds.
3- Control variables based on the adverse effects of novel entities on other Earth systems:
A- Disturbance to biodiversity by plastic pollution.
B- Toxicity of chemical pollutants.
Therefore, researchers are still trying to determine a quantifiable control variable. However, some propose (5) that it is safe to assume that this PB process has transgressed the safe operating space due to the fact that we cannot keep track of the scale of the production and the adverse impact of these pollutants.
Novel Entities – Interactions with other processes
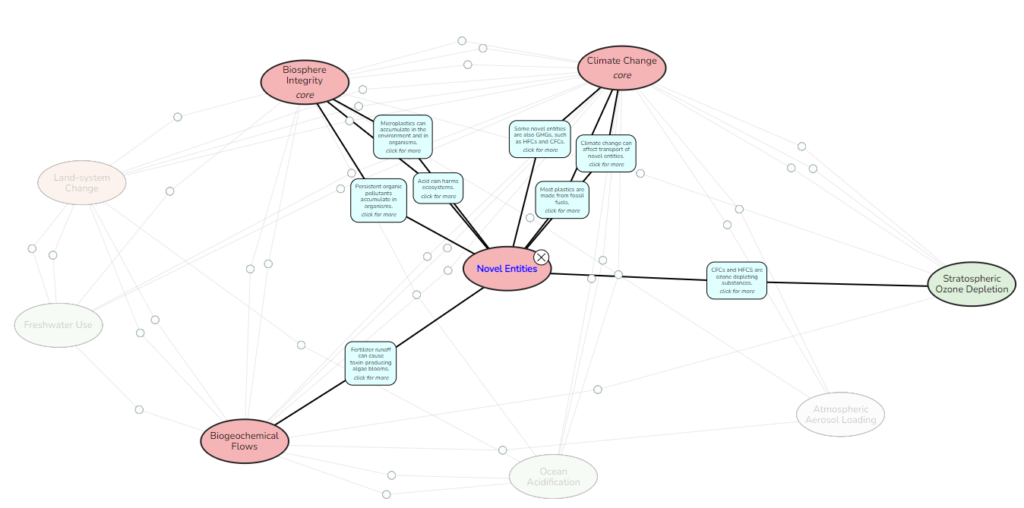
Atmospheric Aerosol Loading
Overview
Aerosols are fine particles suspended in the atmosphere. Naturally occurring aerosols include fog, dust, and volcanic ash. Anthropogenically emitted aerosols such as sulphates, nitrates, black carbon, and CFC are derivatives of emissions from the burning or processing bio and fossil fuels. These particles in the atmosphere interact with the incoming and outgoing solar and infrared radiation through scattering, absorption, and reflection processes. These interactions impact the climate system by altering cloud formation, reflectivity, and persistence, changing monsoons’ temporal and spatial distribution, and altering the precipitation patterns over the region outside the monsoon season. The underlying geophysical processes governing the interactions between the atmospheric aerosol loading, climate system, and monsoon patterns are complex.
The other impact of anthropogenic atmospheric aerosols is on the health of humans and other organisms. In addition, these air pollutants are harmful to the entire biosphere system, affecting genetic and functional diversity.
Atmospheric aerosol loading was primarily chosen as a component of the PB framework due to its intense and complex interaction with the climate system’s global- and regional-scale atmospheric circulation. Depending on these particles’ chemical composition, altitude, and spatial distribution, understanding and quantifying the interactions between Atmospheric Aerosol Loading and other PB processes are highly complex.
Control Variables
The revised version of the planetary boundary framework published in 2015 (3) proposes a control variable, boundary and threshold for the regional ocean-atmospheric circulation in the context of a south Asian monsoon study. The proposed control boundary was Aerosol Optical Depth (AOD). I refer the reader to the source (3- and references therein) for further detail.
As mentioned earlier, due to the complexity of interactions and the variety of these particles, researchers are still working on a quantifiable global control variable with boundary and threshold.
Atmospheric Aerosol Loading – Interactions with other processes
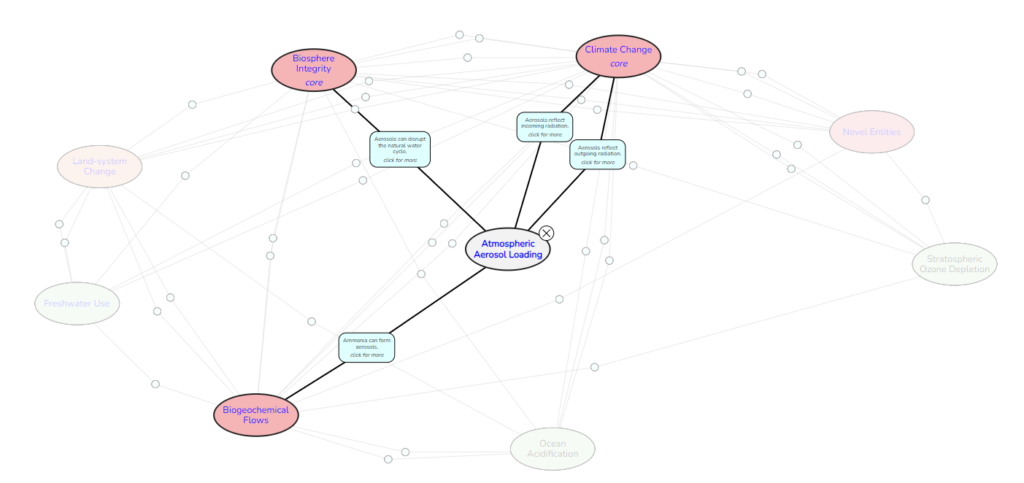
Conclusion
The primary purpose of developing the planetary boundary framework was to provide a scientific standard for humanity to reduce, control, and eliminate the anthropogenic adverse effects that the Earth system processes cannot regulate. The original and other developing frameworks aim to provide a guideline for governance, industries, and business models to operate within environmentally safe parameters. The guidelines are there for all of us to set guard rails and not drive ourselves over the cliff. These environmental and planetary models and frameworks are provided to ensure we continue developing and thriving far into the future, ensuring the quality of life for future generations without compromise.
The data-driven predictive models depict a grim future for humanity at the rate we are currently and persistently harming the Earth systems; however, when we look around and see our children, we must summon all hope and mobilize all our resources to ensure the quality of life for them is not compromised.
We have a lot to fight for. We have the younger generations in front of us to fight for. We are responsible for cleaning up the mess we have created and reversing the adverse effects of multi-generational incompetence and exploitative practices.
It is time to regenerate and restore. Time to adopt net positive strategies.
Payman Janbakhsh, Ph.D.
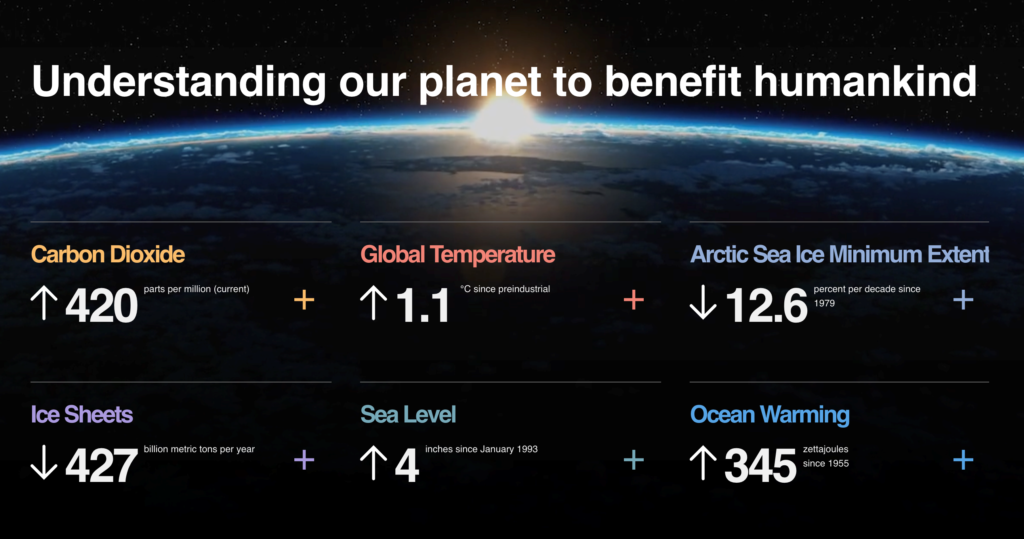
References
(1) Rockström, Johan, et al. “Planetary boundaries: exploring the safe operating space for humanity.” Ecology and society 14.2 (2009).
(2) Rockström, Johan, et al. “A safe operating space for humanity.” nature 461.7263 (2009): 472-475.
(3) Steffen, Will, et al. “Planetary boundaries: Guiding human development on a changing planet.” Science 347.6223 (2015): 1259855.
(4) Biermann, Frank, and Rakhyun E. Kim. “The boundaries of the planetary boundary framework: a critical appraisal of approaches to define a “safe operating space” for humanity.” Annual Review of Environment and Resources 45 (2020): 497-521.
(5) Persson, Linn, et al. “Outside the safe operating space of the planetary boundary for novel entities.” Environmental science & technology 56.3 (2022): 1510-1521.
(6) Mace, Georgina M., et al. “Approaches to defining a planetary boundary for biodiversity.” Global Environmental Change 28 (2014): 289-297.